Faculty Spotlight: Jan Christoph, PhD
Using AI to Envision Cardiac Electrophysiology
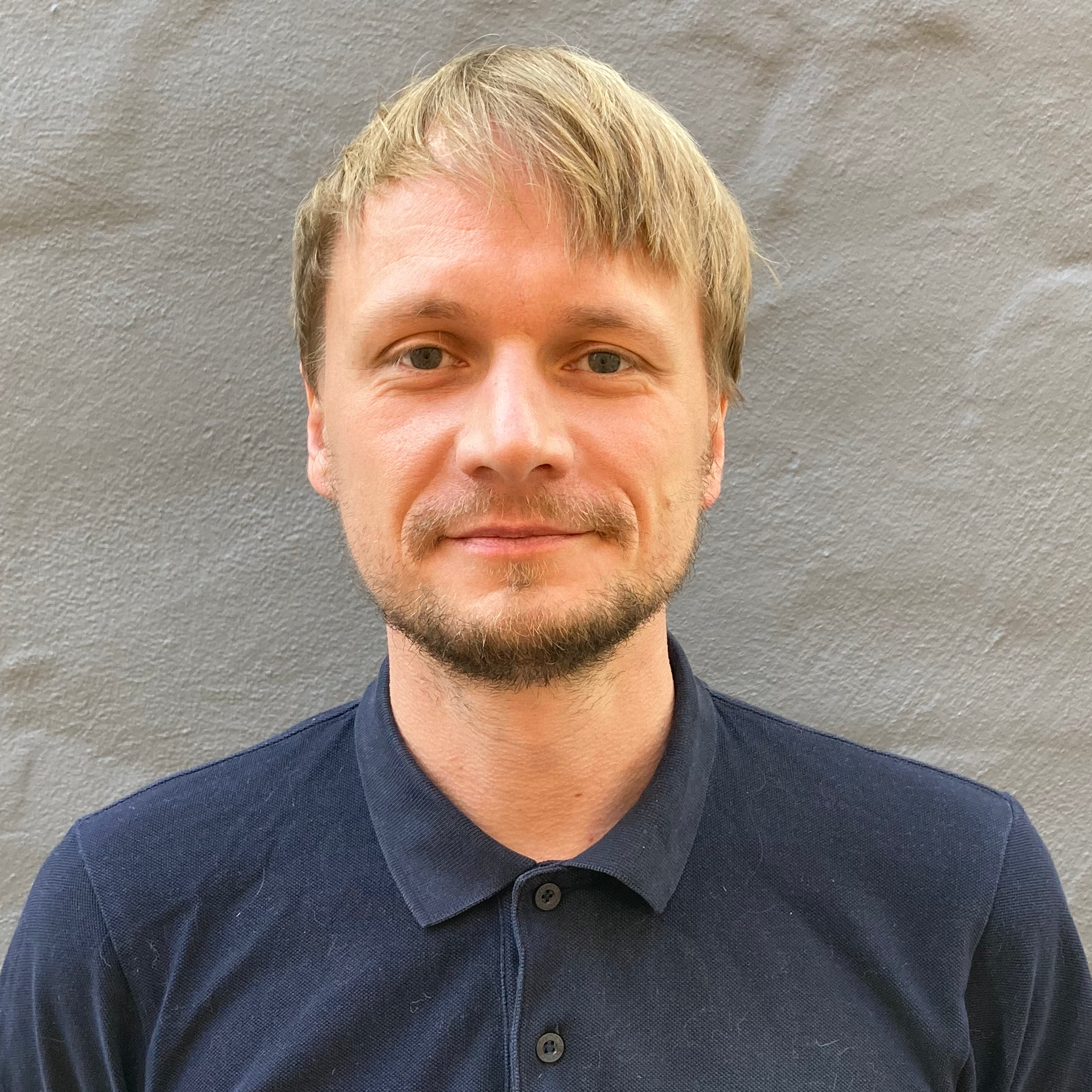
If you’ve ever watched a flock of starlings in the California Delta and marveled how thousands of individual birds could move as one, you’re not alone.
“There are many examples in nature where an individual unit doesn’t have knowledge about the behavior of the entire system, and yet together the individual units act as one, as if they had this knowledge,” said Assistant Professor Jan Christoph, PhD, who holds a doctorate in the physics of biological and complex systems. “This phenomenon is called emergent dynamics, which arise from a set of simple rules such as birds keeping their distance from neighboring birds and trying to fly at the same speed and in the same direction.”
In his studies he became fascinated by the way the human heart’s five billion cells interact with each other in similar ways, working together to pump blood. “One of my special interests is, how do the many heart muscle cells coordinate the heartbeat?” said Dr. Christoph. “How do they communicate and synchronize together to act as one larger phenomenon that emerges on a much larger scale? An individual heart cell doesn’t really know what all the other cells in the heart are doing. However, neighboring cells signal each other via tiny [electrical] currents.” When a heart muscle cell accumulates enough electrical charge to reach a certain threshold, it causes the cell to release a burst of electrical activity known as an action potential. “Those action potentials initiate cellular contractions, which, on the organ scale, form the heartbeat,” he said.
In 2021, Dr. Christoph was recruited to the UCSF Division of Cardiology, and is also cross-appointed in the UCSF Department of Bioengineering and Therapeutic Sciences. He and his team combine bioengineering, artificial intelligence (AI) and physics to find better ways to diagnose and treat heart disease. In particular, they employ a type of AI known from the field of computer vision; this allows the computer to “see” information in the form of pictures, videos and other visual input, and to recognize, interpret and make predictions based on that information. Applying computer vision to better understand heart function inspired the name of his group – the Cardiac Vision Laboratory, which is based in the Cardiovascular Research Institute (CVRI) on UCSF’s Mission Bay campus.
Marrying Electrophysiology with Tissue Mechanics
Although the heart is a complex system, like all physical matter it follows the laws of physics. Dr. Christoph’s team strives to better understand how electrical waves and the resulting muscle contractions coordinate the heartbeat and behave during heart rhythm disorders. They recently developed a novel way to record the electrical signals, also known as action potential waves, which move through the heart and cause it to contract.
Since the 1970s, researchers have been able to “see” these action potential waves by using voltage-sensitive fluorescent dye which is injected into the heart and then imaged using high-speed cameras. The dye allows them to visualize electrical waves moving through heart tissue in real time. However, it has been impossible to image a beating heart using fluorescence imaging, because that movement created “motion artifacts.” There are several contributing factors to this complex problem.
First, unlike neurons, heart cells are constantly in motion, so the physical location of each cell is always changing. That makes it difficult to determine whether a specific cell is electrically activated or not, let alone billions of them at a time. Second, the brightness of the fluorescent dye only changes slightly when it is electrically activated versus resting – a change that is too subtle for the human eye to notice. Third, the intensity of the light on the heart tissue isn't completely uniform; it varies depending on the angle and distance from the light sources, and of course those factors are constantly in flux because the heart is always moving. These and other factors create so much visual “noise” that it was impossible to accurately record action potential measurements from beating hearts. Another limiting factor was that video cameras were so expensive that most labs could only afford one or two, if any.
To get around motion artifacts, most researchers use a compound called Blebbistatin, which inhibits the heart’s contractile motion while leaving the electrical impulses intact. Blebbistatin allows researchers to track how electricity flows through the heart without the artifacts caused by actual heart movement. However, it also prevents them from seeing how electrical activity relates to tissue mechanics – a vitally important part of understanding the physiology of the heart.
Dr. Christoph’s lab developed a fresh approach, enabled by a reduction in the cost of high-speed video cameras, increased computer processing power, as well as advances in AI and computer vision. His lab developed an experimental setup for the imaging of intact, isolated hearts. It includes 12 high-speed cameras, a 3D ultrasound system, and a soccer ball-shaped imaging chamber with many small windows through which the imaging is performed with the 12 cameras. The imaging chamber is also equipped with dozens of LED lights on the inside to excite voltage- or calcium-sensitive fluorescent dyes. When they place an isolated, beating animal heart at the center of the imaging chamber, it is more evenly illuminated, and the cameras and ultrasound imaging system capture high-resolution imaging data of the heart from different angles.
They also developed novel computer vision algorithms to perform post-processing of video data. Since multiple cameras captured each part of the heart from different locations, by combining the images the researchers in his lab could reconstruct the 3D shape and motion of the heart and measure electrical waves in 3D. At the same time, the “noise” created by motion artifacts could be significantly reduced. They recently released open-source software (called “optimap”) to enable other scientists to employ this same approach.
“These computer vision algorithms can track, identify and stabilize motion, which is a game changer in our field,” said Dr. Christoph. “For the first time, we have the tools with which we can study both the heart’s motion and its electrophysiology at very high spatial resolutions. We can correlate these electrophysiological measurements with tissue mechanics. For instance, how do the heart’s ventricular chambers deform in response to these electrical wave patterns? Our recent preprint is the first demonstration that we can image tissue strain as well as electrical activation across the entire ventricular surface at very high spatial resolutions. As far as we know, we are the first lab to show that this can be done.”
Dr. Christoph hopes to refine their algorithms to more accurately measure the flow of electricity through the heart. In addition to learning more about how healthy hearts function, he looks forward to applying this approach to diseased hearts. “I think this is a powerful tool to study many different cardiac pathophysiologies,” he said. “For instance, there are models of cardiac arrhythmias that we could reproduce to study the interactions between tissue mechanics and electrophysiology. This will involve studying calcium, which is involved in cell contraction and released from cellular storage compartments upon electrical activation of the cell.”
Understanding the Feedback Loop between Electrics and Mechanics
Traditionally, many cardiovascular scientists and clinicians either specialize in cardiac electrophysiology – using catheter mapping or electrocardiography to understand what goes awry with the electrical properties of the heart – or mechanics, using imaging modalities such as echocardiography or cardiac magnetic resonance imaging (MRI) to study abnormalities in the heart’s structure and mechanical function. Each field is very complex, and it is challenging to develop and maintain expertise in just one of these subspecialties, let alone both.
Dr. Christoph hopes his research will contribute to more interactions between the two fields, and a better understanding of how these two aspects of the heart’s physiology intertwine. “Disease may originate from one of the two components, but it will affect the other as well, because everything is coupled,” he said “In the future, it would be great if these two fields would merge. With computational modelling and the integration of electromechanical data, I think this can be achieved.”
Dr. Christoph described a phenomenon called electro-mechanical feedback. “On the one hand, the action potential triggers contraction, but the contraction and deformation associated with it can affect mechano-sensitive ion channels, which then in turn influence the action potential,” he said. “There is a feedback loop between electrical activation and mechanical deformation. That’s something we would like to study more.”
Going back to his fascination with emergent phenomena, Dr. Christoph noted how these two mechanisms connect every cell in the heart with all the other cells. “In very abstract terms, we describe each cardiac cell as a ‘nonlinear oscillator,’” he said. “The five billion heart cells are many electrically and mechanically coupled nonlinear oscillators. On the one hand, they pass on their electric excitation to neighboring cells. That’s why you see these electrical wave phenomena in the heart.”
The second mechanism is elasticity. “Imagine a balloon,” said Dr. Christoph. “If you squeeze the balloon on one side, you can feel it on the other side because everything is stretched towards the part that contracts. The cells in the heart sense mechanical activity from further away. As one part of the heart contracts, the opposite side is strained due to that contraction. The electrical oscillators become modulated by the mechanics. All of this is enormously complex, and we aim to study and dissect this complexity using quantitative tools such as computer modeling and imaging. Of course, this cannot be addressed by a single lab alone. This is really a collaborative effort across many labs and generations of scientists who have built and are building this knowledge.”
Using Imaging Data to Predict Electrical Activity
Dr. Christoph’s laboratory is currently funded by the National Institutes of Health (NIH) to develop a novel imaging approach for non-invasive imaging of heart rhythm disorders using ultrasound imaging. Dr. Christoph received the NIH Director’s New Innovator Award in 2022, a prestigious multiyear grant which supports exceptionally creative early-career investigators who pursue high-risk, high-reward projects. “Together with clinicians, we want to study whether we can use ultrasound or MRI imaging data, which is structural data, to compute the electrophysiological activation patterns that must have caused those deformations that we see during heart rhythm disorders,” he said. “I hope that in a few years, we’ll have an answer to whether this is possible in patients or not.”
The need to improve diagnostics in cardiac electrophysiology motivates Dr. Christoph’s quest to forge this novel imaging tool, which holds the potential to visualize heart rhythm disorders instantaneously and entirely non-invasively. The current way of detecting the heart’s electrical activity requires an electrophysiologist to thread a catheter up through a patient’s blood vessel into the heart, then use an electrode integrated into the catheter to measure electrical activity point-by-point in the heart. While this type of electrical mapping can identify the location of abnormal electrical activity which causes irregular heart muscle contractions, it can be time-consuming and only records activity on the surface of the heart. It would be ideal to have a way to visualize the three-dimensional electrical wave patterns at play in the heart, to better diagnose and treat arrhythmias.
In addition to developing approaches like the soccer ball-shaped imaging chamber, Dr. Christoph’s group is also a computational lab with expertise in simulating phenomena like the heart’s electrical and mechanical activity. “One question we are trying to answer is, can we develop an AI that can predict the electrical map of a heart from the structural ultrasound image?” he asked. His group is tackling this problem using computational methods and deep learning.
Deep learning is a subfield of AI, in which so-called “deep neural networks” are trained on data which allows them to recognize patterns and fulfill tasks based on information they extract. “Deep neural networks can recognize objects in a visual scene,” said Dr. Christoph. “In our case, they learn to recognize certain patterns of motion, and associate these patterns with electrical activation patterns.”
Deep learning takes vast amounts of input, which the network analyzes to produce output. It requires a lot of training data upfront, learning from it in a trial-and-error process until it gets better at producing high-quality output. For example, a programmer may feed in thousands of pictures of cats to a deep learning algorithm. The algorithm breaks the picture into small pieces – learning to identify shapes associated with a cat’s eyes, nose, ears, whiskers, face, paws, tail, fur, and other parts. Then it puts all the elements together to decide how likely a new picture features a cat.
To try to predict a heart’s electrical activity from its mechanical activity, Dr. Christoph’s lab first used a computer simulation model to generate a training set with 100,000 paired examples of action potential waves and mechanical motion. They randomly varied the geometries of each model heart, changing up the size of the chambers, thickness of the heart wall, and adding scars in various locations to some examples, similar to those found in patients with myocardial infarcts. “Just like each patient is different, we wanted to add as much diversity to these training datasets as possible, so this network has seen all possible cases and combinations,” he said.
The deep neural network analyzed all this data. “After seeing 100,000 examples, the network was like, ‘Okay, I think I understand the mapping between the two,” said Dr. Christoph. “We didn’t know if it would work, or if there was even a solution to this problem, but the fascinating thing is that a neural network is able to do it.” When the researchers fed in other examples of just the mechanical activity, the deep learning network was able to predict the electrical wave activity that caused that specific pattern of heart contraction, very accurately and reliably. “No matter how complicated the deformation was, this network could figure it out,” he said.
The numerical work was recently published in a preprint. To prepare the deep learning network to work with real-world data from patients, Dr. Christoph’s lab has also trained it on data that was partially obscured or noisy. “The network learns to ‘see’ structure in noisy images and estimate noise so it can remove it and get to a less noisy version of that image,” he said.
After establishing this numerical proof-of-principle, the lab is currently working to reproduce the results with animal model hearts in their imaging setup. They plan to feed ultrasound and fluorescence recording data from the imaged isolated hearts into the deep learning algorithm, see what the algorithm predicts based on the ultrasound data, and then check that prediction against the actual 3D electrophysiological activation patterns that they are now able to record using their new imaging setup. If the team succeeds, they will move on to clinical data, such as using heart ultrasounds to predict patients’ electrophysiological activity.
From Superficial Measurements to 3D
Dr. Christoph’s lab is also tackling a related problem: how to use imaging data of the heart’s surface to visualize not just the electrical activity on the surface of the heart, but the 3D electrical dynamics moving throughout the whole heart muscle. It is a bit like the difference between dropping a pebble into a swimming pool and watching the ripples move outwards on the surface, compared with being underwater and getting to see how the pebble’s wake disrupts the water both on the surface and throughout the pool.
“Until now, even though we performed high-resolution measurements, we couldn’t visualize what was happening below the surface, because the heart is opaque,” said Dr. Christoph. “At the same time, we know these waves adhere to certain rules. Each cell behaves like a nonlinear oscillator. Because it receives input from neighboring cells, we expect to see certain patterns in these so-called 3D reaction-diffusion systems. These patterns are well-known in the physics and mathematical biology community.”
Again, Dr. Christoph and his team used deep learning networks to try to solve this problem. Using computer simulations, they started by generating 3D wave patterns, then shaved off just the very top layer of this pattern to create a 2D image. They fed many of these related 3D and 2D image pairs into the algorithm to train it, then started feeding in just the 2D images of other examples. “This neural network, based on its training, was able to anticipate what the 3D structure must have been,” he said. “The network is able to do this because it has seen 100,000 examples and learned that this 2D pattern must belong to that 3D pattern. There are still some differences, but overall, it comes up with a relatively good reconstruction of this volumetric wave pattern. We hope we can fill in the missing gaps with such techniques in the future.”
Although AI holds much promise, Dr. Christoph is mindful of its imperfections. “People often use AI, but they can’t always guarantee that it will come up with accurate solutions,” he said. “We hope to help address questions about how AI can be used responsibly in cardiology, and what measures need to be taken to limit its risks when used for diagnostics or to guide therapies. There are many flavors of AI, and potential risks depend on its use. I anticipate that we will move beyond purely data-driven AI, and will use approaches which incorporate physical laws and take into account knowledge about the behavior of the biology. I think in the future, AIs will be constrained by such hybrid methods to help make sure that what they come up with is accurate and valid.”
At the moment, Dr. Christoph’s lab is one of just a handful worldwide that is conducting research at the intersection of these particular fields. “This is a niche subject,” he said. “Our projects touch on many different aspects of imaging, physics, computer vision, AI, dynamical and complex biological systems, mechanical engineering, and the electrophysiology of cells and tissues. UCSF is one of the few places in the world where you can do this kind of interdisciplinary research.”
Eager to Collaborate with Clinicians
Dr. Christoph grew up in Clausthal-Zellerfeld, a small German college town. He became intrigued with physics in high school. “The most fascinating topic was that you can describe and predict a process with mathematical equations, and use quantitative methods to better understand how a certain phenomenon arises,” he said.
He earned his diploma – the equivalent of a bachelor’s and master’s degree – in physics from the University of Göttingen in Germany, where he also completed his PhD in the physics of biological and complex systems. He was a postdoctoral scholar at the Max Planck Institute for Dynamics and Self-Organization in Göttingen, then served as a tenured scientist in the Department of Cardiology and Pneumology at the University Medical Center Göttingen before joining the UCSF Division of Cardiology faculty in May 2021.
He was drawn to UCSF because of its outstanding combination of basic and clinical research. “I was lucky enough to find this position where I have the chance to work with one foot in the clinical setting, collaborating with cardiologists at UCSF, and on the other hand I’m considered a basic scientist who is part of the CVRI, together with other basic scientists who study very fundamental biological questions,” said Dr. Christoph. “I’m looking forward to working with so many great clinician-scientists and scientists. There are a lot of interesting questions where this hybrid of computational-clinical-wet lab approach could be very fruitful.”
For example, he hopes to collaborate with cardiac electrophysiologists, as well as those who specialize in forms of congenital heart disease such as hypertrophic cardiomyopathy (HCM), in which an abnormally thickened heart muscle can cause life-threatening arrhythmias. “UCSF is really collaborative and supportive, and I feel a very positive attitude from people,” said Dr. Christoph. “People have good intentions and are here to improve healthcare and cure diseases. They are trying to develop things that can improve people’s lives. It is a very interesting community to be part of.”
“Jan’s work is a novel way to look at how abnormal heart rhythms, such as ventricular fibrillation, are generated and sustained,” said Jeffrey Olgin, MD, Ernest Gallo-Kanu Chatterjee Distinguished Professor in Clinical Cardiology and chief of the UCSF Division of Cardiology. “He is developing and applying very innovative approaches to mapping these arrhythmias, and applying machine learning techniques to make them more widely applicable. We hope that some of these methods and tools will allow us to better identify the origins of these abnormal heart rhythms so that they can be ablated, or cauterized with a catheter.”
“Jan brings a unique combination of scientific expertise and rigor, technical expertise and computational skills to the Division of Cardiology and broader UCSF cardiovascular research environment,” said cardiologist Geoff Tison, MD, MPH, whose own lab focuses on using machine learning and large-scale epidemiologic and clinical research methods to achieve goals in disease prevention, prediction and disease phenotyping. “There are countless collaborations that are now made possible by virtue of the unique expertise he brings to the division.”
Picking the Important Questions
Dr. Christoph enjoys working with the trainees in his lab, who range from undergraduates to postdocs. “In the past few years there has been so much progress in the field of AI, and it’s been very exciting and rewarding to discuss these developments within our group,” he said. “I feel very lucky to be able to work with such smart and talented people. My trainees constantly bring in fresh ideas and knowledge, and interacting with them is a really nice part of my work. I try to give people the support and structure they need to succeed, while also providing the freedom to pursue their interests and develop their skills. Success is hard to predict, but I believe that people will become successful when you let them do what they are good at and enjoy.”
Because his group is breaking new ground, there is a lot of trial and error in their approach. “Often, we throw methods at a problem and see how well they work,” said Dr. Christoph. “If they don’t work, we try a different one until we hit on one that shows promise. It takes a little bit of intuition to not waste too much time on any one thing, and to pick the right method and ask the right questions. My job is to make sure that we are sorting out the important questions from the less important ones.” In the coming years, he hopes to recruit more clinical trainees to his lab, including medical students, residents, fellows, and MD/PhD students.
“I hope that one day our research will help patients,” said Dr. Christoph. “A dream of mine is that heart rhythm disorders could be visualized instantly, and that an algorithm could immediately recognize a particular problem in patients and intervene. Knowing that these new computational methods will eventually help patients is really great.”
Outside of the lab, Dr. Christoph enjoys swimming, is learning to surf, and was a longtime guitar player, bassist and singer in several punk and metal bands.
- Elizabeth Chur