Faculty Spotlight: Vasanth Vedantham, MD
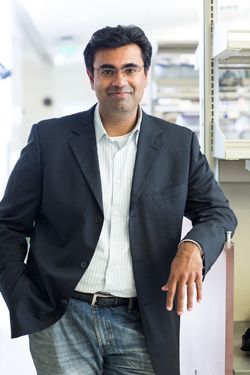
"The heart is a truly amazing organ," said cardiac electrophysiologist Dr. Vasanth Vedantham. "In a typical person’s lifetime, it beats about 2 billion times, and with each heartbeat a precisely timed sequence of events has to occur with very little margin for error. If you don’t have a heartbeat for several seconds, you’ll begin to lose consciousness, and if that lasts for more than a couple of minutes, you could have irreversible brain damage."
Dr. Vedantham’s research focuses on this very fundamental biological process, one that most of us are fortunate enough to take for granted: the basic mechanisms that allow the heart to generate and maintain its own rhythm. "It’s a question that historically attracted a great deal of scientific interest, but hasn’t received as much attention recently, because now when the body’s natural pacemaker fails, we just implant an artificial electronic one," said Dr. Vedantham. "But I think developing a deep understanding of how the system is designed is very important, and may lead to completely new kinds of treatments that could make electronic pacemakers obsolete.
The heart has evolved a sophisticated "wiring system" that controls how a heartbeat originates and spreads throughout the entire organ. The human heart has its own built-in pacemaker cells, which are concentrated in an area called the sinoatrial (SA) node.
"The vast majority of heart muscle cells are electrically quiescent until they receive a signal to beat, but pacemaker cells generate their own electrical signals – they don’t need a signal from any other cell," said Dr. Vedantham. "However, one pacemaker cell on its own can’t generate enough current for the entire heart, so there are many thousands of them in the SA node. Each time a heartbeat initiates, a few pacemaker cells may fire, then more and more until you have a wave of electrical activation that exits the SA node and produces enough current to trigger a heartbeat."
When he started his postdoctoral research training several years ago in the lab of Dr. Deepak Srivastava at the Gladstone Institute of Cardiovascular Disease, Dr. Vedantham was surprised to learn that a number of very fundamental questions about the SA node have yet to be answered. "How do pacemaker cells differ from regular heart muscle cells?" asked Dr. Vedantham. "How do they become organized and interconnected in an optimal arrangement for producing a stable heart rhythm? Why and how does our resting heart rate change over time, such as in normal aging and with athletic training? How is this different from people who have lower heart rate from SA node disease or other types of heart disease? Is SA node disease a problem with loss of pacemaker cells, a problem with the way they are arranged, or something going wrong within individual pacemaker cells? I quickly became fascinated by these questions and decided to try to tackle them experimentally in the lab."
Zooming in on Pacemaker Cells
To begin to address these questions, Dr. Vedantham knew he would need to be able to isolate large numbers of intact pacemaker cells, something that is essentially impossible to do from living humans. Fortunately for Dr. Vedantham, the basic structure of the SA node is conserved in much of the animal kingdom. "It’s remarkable that animals as large as a blue whale, whose resting heart rate is only 10 beats per minute (once every six seconds), and as small as a mouse, whose resting heart rate is 600 beats per minute (ten times every second), have pacemaker cells and an SA node that are pretty similar to what we have," said Dr. Vedantham.
"This means that we can apply cutting-edge technologies in molecular biology to genetically tractable animal models in order to study pacemaker cells," he said "As we learn more from such systems, my hope is that we will be able to dispense with animals entirely and derive pacemaker cells directly from human stem cells."
Even using animal model systems, a major research challenge that Dr. Vedantham has faced is isolating pacemaker cells from the rest of the heart so he can better study them. Pacemaker cells are interspersed with different kinds of cells in the heart, including the force-generating cardiomyocytes, which form the muscular walls of the heart; fibroblasts, which make the scaffolding of the heart; nerve cells; and endothelial cells, which line the interior of blood vessels and heart valves. Even in the SA node, pacemaker cells make up a surprisingly small fraction of the total number of cells (about 1 to 2 percent). "So even if you excise the entire SA node from the heart for further study, only a tiny portion of the material you end up with will consist of pacemaker cells," said Dr. Vedantham.
To overcome this hurdle, Dr. Vedantham obtained a genetically modified mouse in which pacemaker cells expressed a fluorescent marker, making them easy to identify under a microscope. He then used a method called laser capture to cut out microscopic clusters of pacemaker cells. By laying a thin sheet of heart tissue on a glass slide and magnifying the image on a computer screen, the fluorescently tagged pacemaker cells show up as long, thin green fluorescent cells, standing out from other heart muscle cells on the slide. By tracing the outline of the green fluorescent region, Dr. Vedantham can direct a laser to cut along that line, a little bit like cutting a cookie from a sheet of dough. "After cutting out the area with the pacemaker cells, we then use laser light to catapult the tissue off of the glass slide and into a collection tube," said Dr. Vedantham.
By collecting such concentrated samples of pacemaker cells, Dr. Vedantham and his group were then able to profile their gene expression networks – identifying which genes are turned on in pacemaker cells but not in other cell types in the heart, which provides clues about which genes are involved in guiding a heart cell to specialize into a pacemaker cell rather than another kind of cardiomyocyte.
One striking finding was that pacemaker cells activated genes that are typically used by nerve cells to wire the brain, regulate the flow of electricity, and establish connections between neurons, suggesting that although pacemaker cells are still heart muscle cells, they have co-opted part of the gene expression program of nerve cells, which allows them to have their unique electrical behavior. The team also collected and analyzed pacemaker cells from mouse hearts at different stages of development, from embryos to adult mice, to learn which genes were activated at different phases.
Dr. Vedantham demonstrated that a gene called Islet-1 – a transcription factor whose function is to control the activation of other genes – is turned on in pacemaker cells, where it activates the genes that endow pacemaker cells with their unique electrical properties. He found that when Islet-1 is genetically removed during SA node development, pacemaker cells become more like regular heart muscle cells, and are less able to initiate the electrical impulses that create a heartbeat.
Abnormal behavior by gene regulatory networks in pacemaker cells, such as the one controlled by Islet-1, may be very relevant to human sinus node disease, which remains the most common cause for permanent pacemaker implantation. When the biology of a heart muscle cell changes, or scarring develops in the heart, the body is less able to regulate the flow of heartbeat-producing electricity through the heart. "That’s why arrhythmias accompany every single form of heart disease, and are often the leading cause of death in various types of heart disease," said Dr. Vedantham.
However, the fact that pacemaker cells can change their behavior in response to stresses or genetic modifications raises the possibility of harnessing these gene regulatory networks in pacemaker cells to treat disease. "I’m interested in testing whether we could influence gene regulatory networks to tell ordinary heart muscle cells to become pacemaker cells, or to tell sick pacemaker cells to act more like robust pacemaker cells," said Dr. Vedantham. "But first we need to understand how those systems are set up. We’re at the beginning of that road."
Deducing Cause and Cure
Dr. Vedantham was born and raised in the Chicago suburbs, where his father was a physician and his mother was an interior designer. He earned a bachelor’s degree in physics and a master’s degree in biochemistry from Yale. He was drawn to the human interaction of medicine, yet maintained a passion for science, which led him to the physician-scientist career path. Dr. Vedantham earned his medical degree, doctoral degree in neurobiology, and master’s degree in philosophy from Harvard. His doctoral research focused on a group of proteins called voltage-gated sodium channels, which regulate electrical conduction across cell membranes and play key roles in the nervous system, the heart, and in other muscles.
After completing his internal medicine residency at Brigham and Women’s Hospital in Boston, Dr. Vedantham then came to UCSF for his cardiology and cardiac electrophysiology fellowships. "I particularly like cardiology because you can think through clinical scenarios in a way that doesn’t just rely on knowing a lot of information – you can often analyze problems and derive solutions from first principles," he said. "And with cardiac electrophysiology, during a single procedure, we are able to stimulate the heart, record the response, deduce what might be causing a patient’s symptoms, then figure out exactly where the abnormal tissue is and eliminate it. It’s very satisfying when you can cure someone of something that’s been plaguing them for years, or even save a life by implanting a defibrillator in a high-risk patient."
In addition to performing cardiac electrophysiology procedures – implanting devices and ablating abnormal heart tissue to prevent arrhythmias from recurring – Dr. Vedantham also has a clinical interest in an uncommon disease called cardiac sarcoidosis, in which clusters of inflamed tissue called granulomas invade the heart muscle, often leading to severe arrhythmias and heart failure. He co-manages these patients with many other sarcoidosis experts at UCSF, including his wife, Dr. Julie Zikherman, a UCSF immunologist and rheumatologist.
Dr. Vedantham is also developing expertise in inherited arrhythmia syndromes, a group of diseases that result from mutations in genes that are important for normal flow of electricity throughout the heart. These diseases are especially tragic, because they can strike young people who seem otherwise completely normal, and sudden death may be the first manifestation of the disease. "When one of these conditions is suspected, we have to use all of our diagnostic tools," said Dr. Vedantham. "These include a careful and detailed patient and family history, electrocardiograms and other imaging tests, as well as newer genetic tests that can determine whether a patient harbors a disease-causing mutation and whether their family members might be at risk." Armed with this information, Dr. Vedantham can counsel patients and their family members on ways to reduce their risk of having serious arrhythmias.
"Arrhythmias affect patients in all age groups and in all walks of life, so one of the great privileges of being a cardiac electrophysiologist is that we get to help patients make really consequential decisions that don’t necessarily have a single ‘right’ answer," said Dr. Vedantham. "We have to take each patient’s particular circumstances into account and facilitate decision-making that fits best with their own goals.
"For example, I often see patients at elevated risk for sudden cardiac death who might benefit from having a defibrillator implanted, an effective treatment that nonetheless requires adjusting to life with a permanently implanted device," he said. "Sometimes, when the risk is very high, the decision is very clear, but more often things are not so clear-cut. And it’s a very different kind of conversation with a young person who might be concerned about the impact on school and athletics, a middle-aged person with dependent children, or an older adult whose main concerns might be avoiding functional limitations and maintaining quality of life. When I have these discussions I am continually reminded that what is most important in a person’s life can change depending on very particular circumstances, and that patients are often faced with the difficulty of balancing competing values to arrive at the best decision. Helping patients get to a place that is right for them is one of the most rewarding parts of my job."
In addition to his clinical work, Dr. Vedantham is a visiting scientist at the UCSF-affiliated Gladstone Institute of Cardiovascular Disease, where he has worked closely with Dr. Srivastava’s laboratory. He is now establishing his own research lab in the Smith Cardiovascular Research Building at UCSF, where he will advance his work on understanding pacemaker cells and the basic biology of arrhythmias.
Outside of medicine and science, Dr. Vedantham enjoys hiking and reading. He and his wife, Dr. Zikherman, have two young children.
– Elizabeth Chur