Faculty Spotlight: Di Lang, PhD
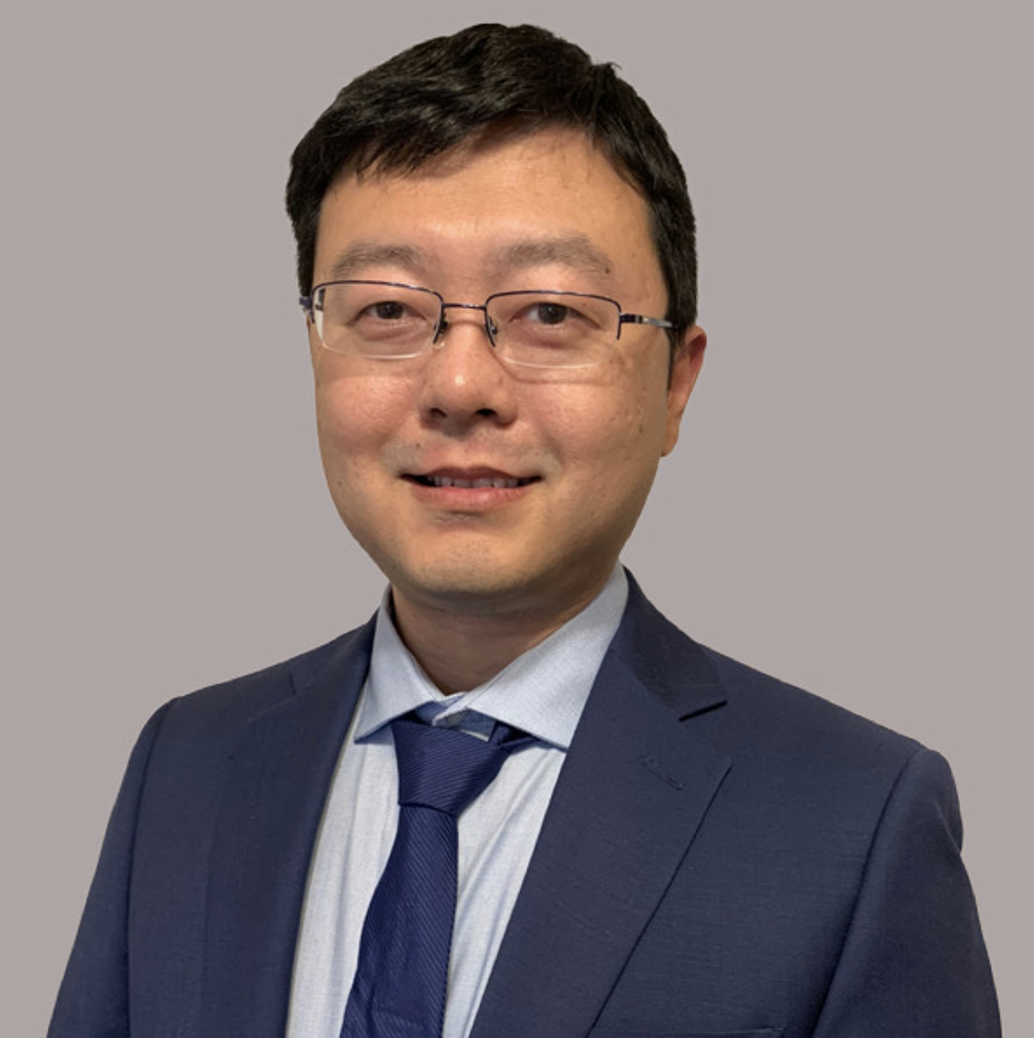
Engineering New Tools for Discovery
As a biomedical engineer, Di Lang, PhD (pronounced “Dee Long”), uses imaging techniques to more precisely calculate and quantify the biomechanics of the heart. In particular, he designs systems to better understand the electrophysiology of individual cells as well as whole hearts. He uses these novel approaches to discover more about what goes awry in diseased hearts that develop abnormal heart rhythms called cardiac arrhythmias.
Dr. Lang was born in China, where his mother was a doctor and his father was an engineer. “My mom wanted me to study medicine, and my dad wanted me to study engineering,” he said with a laugh. “Then they discovered biomedical engineering, which combined everything they wanted! I was fascinated with using engineering thinking to solve biomedical problems and help people.”
For example, Dr. Lang created a platform that allowed him and his collaborators to simultaneously measure electrical signals in single heart muscle cells, also known as cardiomyocytes, as well as visualize the interactions among neighboring cells. “There’s a lot of research that’s focused on single [cardiomyocyte] cells, but when we isolate those cells, they lose their physiological environment,” he said. Because electrical signals flow through the heart in waves, it’s important to understand not just individual cells, but how they interact with each other.
Using a technique called confocal microscopy, Dr. Lang uses a fluorescent dye that attaches to cardiomyocytes. Then with a super-resolution microscope, he can visualize the 3D structure of a single living cell, and can even obtain sharp, clear images of structures within that cell. He also employs a technique called optical mapping, which allows him to visualize how electrical signals flow along the surface of the heart. “By doing optical mapping on the tissue level, we can visualize the whole heart and see how electrical signals propagate, including where those signals are faster or slower,” he said. “We can also see if there is irregular generation of electrical signals.”
When the Safety Net Breaks: Arrhythmia in Heart Failure
In the normal heart, electrical signals originate in an area of the right atrium called the sinoatrial (SA) node, sometimes known as the pacemaker of the heart. That electrical wave then flows down through the upper chambers of the heart, known as the atria, and into the lower chambers of the heart, known as ventricles – causing the heart to contract in a smooth, coordinated manner to pump blood efficiently.
However, sometimes there are rogue cardiomyocytes in other areas of the heart that produce abnormal electrical signals. Instead of waiting to receive electrical signals originating in the SA node, these rogue cells produce their own electrical signals. “If there’s only one cell generating abnormal signals, it’s not enough to activate the whole realm,” said Dr. Lang. The heart has some built-in protective mechanisms that he is trying to better understand.
One of those mechanisms is called cell-to-cell coupling. It allows neighboring heart cells to transfer ions like sodium and calcium between one another through pumplike channels, in a phenomenon called gap juncture conductance. These channels function like gates, and receiving electrical signals can make them open or close. In a normal heart, neighboring cells have good communication with one another – healthy cell-to-cell coupling. So if a lone rogue cell inappropriately emits its own electrical signal, its neighbors serve as a buffer, each absorbing a small amount of excess electricity that is not enough to set off a chain reaction.
“Just one rogue cell is not dangerous if it’s surrounded by a lot of normal cells,” said Dr. Lang. In fact, he and his colleagues used confocal imaging to find that about 1 percent of cardiomyocytes emit these rogue signals, but the remaining 99 percent of law-abiding cells can absorb that aberrant activity without causing the heart to develop arrhythmia.
“The problem is, we do sometimes see abnormal electrical activity in the heart,” said Dr. Lang. “So how many cells are needed to generate enough signal to activate the surrounding cells? That’s the question we’re asking now.” For example, using confocal microscopy, they found that in an animal model of heart failure, rogue cardiomyocytes make up about 10 percent of total cells.
But the number of rogue cells is not the only factor contributing to cardiac arrhythmias. Dr. Lang and his collaborators found that the cell-to-cell coupling in the heart failure model was poor. In other words, not only were there more rogue cells, but there was much less of a buffer surrounding those abnormal cells, since the neighboring cells were less able to absorb abnormal electrical signals. He also has found that in a heart failure model, the disease process is dynamic. With time, abnormal electrical activity spills over from isolated rogue cells; even though there is poor cell-to-cell coupling, there is so much aberrant electrical activity that it eventually causes neighboring cells to start contracting at inappropriate times, perhaps because these cells are themselves poorly coupled to the rest of the heart.
Dr. Lang hypothesizes that the original rogue cells, plus these neighboring “helper” cells which are starting to malfunction, could together form clusters called triggering islands. These can lead to what is known as ectopic beats, or “extra” heartbeats. Most people have occasional ectopic beats, but if they become frequent, they can cause problematic arrhythmias.
By running mathematical simulations, Dr. Lang also found that the more the cell-to-cell coupling ability has been compromised, and the larger that diseased area is, the fewer rogue cells are needed to set off arrhythmias. “The question of how many rogue cells are needed to cause arrhythmias depends on how robust the safety net of cell-to-cell coupling is, as measured by the quality of gap juncture conductance,” said Dr. Lang. “The better the gap juncture conductance, the more abnormal electrical activity it can absorb, so you’d need more rogue cells to trigger an arrhythmia. If there is a small area of reduced gap juncture conductance, you’d need fewer cells, because the buffer isn’t as good. But the worst of all scenarios is poor gap juncture conductance over a large area – then you’d need very few rogue cells to create an arrhythmia.”
That also suggests that to treat the problem, addressing the rogue cells themselves as well as improving gap juncture conductance might be therapeutic targets to prevent arrhythmias from occurring.
Shifting Gears: Pinpointing Different Pacemaker Clusters
Dr. Lang has also helped discover more about the physiology of the SA node – the body’s natural pacemaker, which in a healthy heart will produce the electrical impulses that initiate each heartbeat. He and others have found that rather than being one fixed spot in the heart, the SA node is actually made up of perhaps a dozen or more clusters of cells, each responsible for initiating a narrow range of beating rates. “Originally, people used to believe that the SA node was only one group of cells that always did the same job, whether the heart was beating faster or slower,” said Dr. Lang. “Later on, people found that the SA node actually moved to different places, depending on heart rate.”
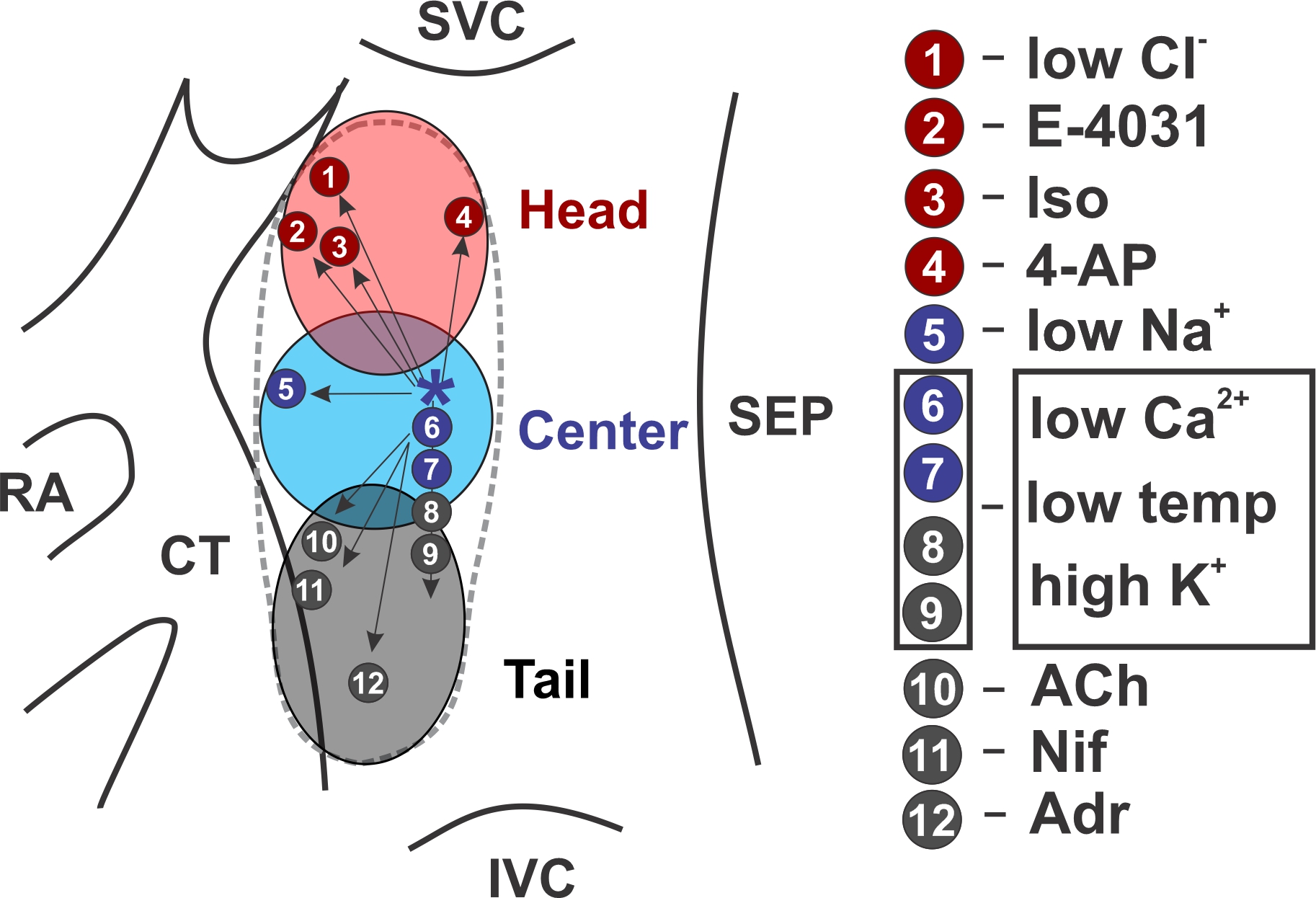
This holds true across many species. One group of pacemaker clusters associated with baseline beating rates – for example, when someone is sitting in a chair – is physically located near one another. (Figure 1) Another group of pacemaker clusters associated with faster beating rates – such as when someone is walking or running – is located above the baseline group. A third group of pacemaker clusters associated with slower beating rates – for example, when someone is meditating or sleeping – is located below the baseline group. Similar to gears on a bicycle, each pacemaker cluster is associated with a specific range of beats per minute, and takes the lead when the body requires that amount of circulating blood.
For years, researchers inferred that the location of the pacemaker clusters was shifting, based on electrocardiogram (ECG) maps which showed that the shape of the P wave changed; that suggested that the place where each heartbeat began varied, depending on heart rate. Later on, researchers used optical mapping to identify the changing location of the initiating pacemaker clusters.
“What I did was create a method that can localize those different cells when they are beating at different rates,” said Dr. Lang. That is allowing him and his collaborators to precisely identify which cluster is active at a given moment, helping them to isolate these clusters and study them one at a time.
This is helping them and others to learn more about the distinct functional and molecular signatures associated with each pacemaker cluster. For example, certain clusters appear to be activated by particular ion concentration levels, neurotransmitters, hormones or medications. “The widely accepted belief is that heart rate changes because of neuron regulation – through sympathetic or parasympathetic stimulation,” said Dr. Lang. “But I believe that there are more complicated responses that regulate our hearts, and that may also generate different pathological conditions. There are likely key proteins or signaling pathways that regulate each pacemaker cluster. In the next couple of years, we hope to better understand which proteins or genes are associated with each pacemaker cluster, and their underlying mechanisms. We want to explore the very complicated regulation of the SA node.”
This could have important implications for better understanding a condition called sinus node dysfunction or sick sinus node syndrome, which is associated with aging as well as some forms of cardiac disease. “People generally lump sinus node dysfunctions together, saying the sinus node is beating too fast or too slow,” said Dr. Lang. “I want to precisely separate different subpopulations of these sinus node cells, because I hypothesize that sinus node dysfunction actually results from damage from different causes, depending on which subpopulation of cells is damaged. The platform I designed helps us distinguish these different subpopulations so we can precisely study each one. These cells are likely pretty similar to one another. There’s only some protein or RNA expression that differentiates each subpopulation.”
Identifying the underlying mechanisms, and what goes wrong in disease, could eventually help cardiologists pinpoint the cause of a specific patient’s sinus node dysfunction, and point to promising avenues for therapy.
Form Follows Function
Dr. Lang has also used his engineering expertise to learn more about the physiology of other subpopulations of cells in the atrium. One key component of cardiomyocytes is a structure called a transversal-axial tubule system (TATS), also known as a T-tubule. These finger-shaped protrusions are rich in ion channels, pumps, and other features which are critical to a heart muscle cell’s ability to contract. The more T-tubules a cardiomyocyte has, the more vigorously it can contract.
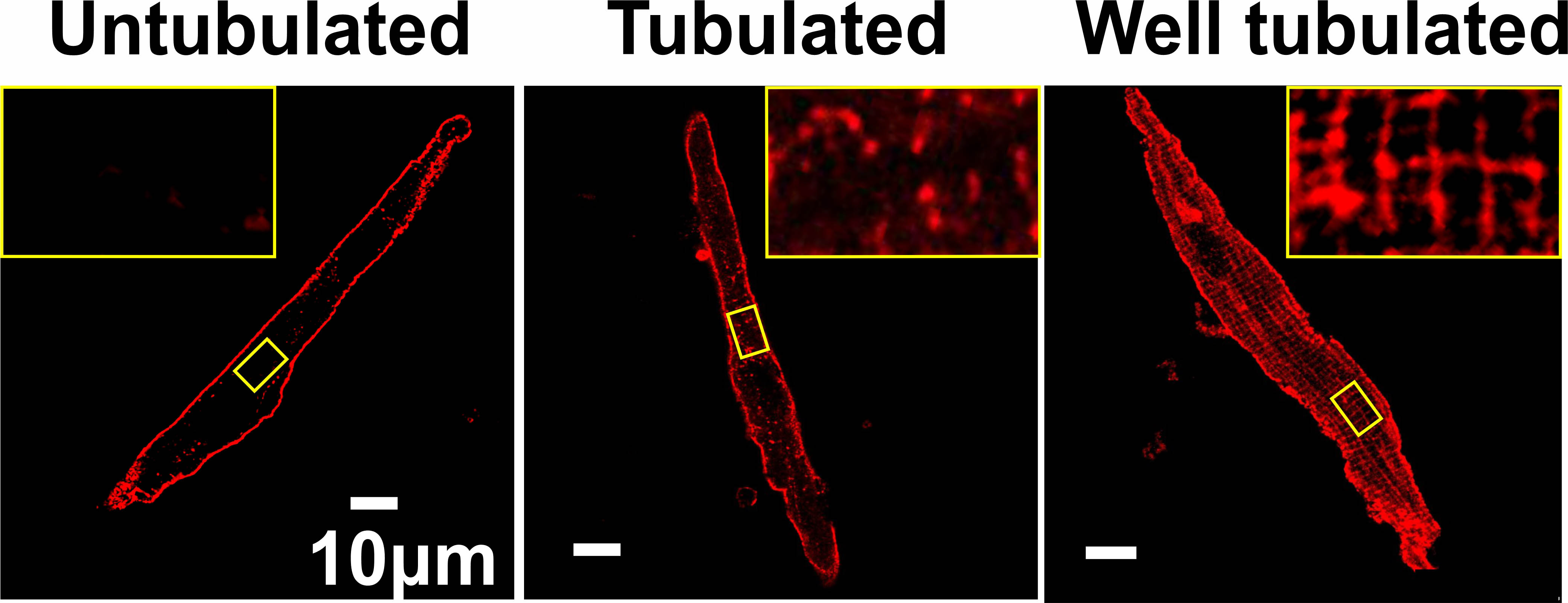
While all ventricular cells appear to have many T-tubules, it’s a different story in the atria. Using high-resolution confocal imaging, Dr. Lang and his collaborators found a wide range of T-tubule density in the right atrium. “Some right atrial cells have a lot of T-tubules, and others had very few,” he said. (Figure 2) “That heterogeneity in their structure is actually linked to the heterogeneity of their functions.”
To conduct this research, Dr. Lang designed a platform to image thousands of individual cells from different parts of the right atrium. He then created a MATLAB image-processing algorithm to count the number of T-tubules in each cell, thereby calculating the T-tubule density. He ensured that the algorithm would count each T-tubule, no matter at what angle it was positioned relative to the camera. “This platform allowed us to analyze thousands of images automatically, something that would have taken us years to do manually,” he said.
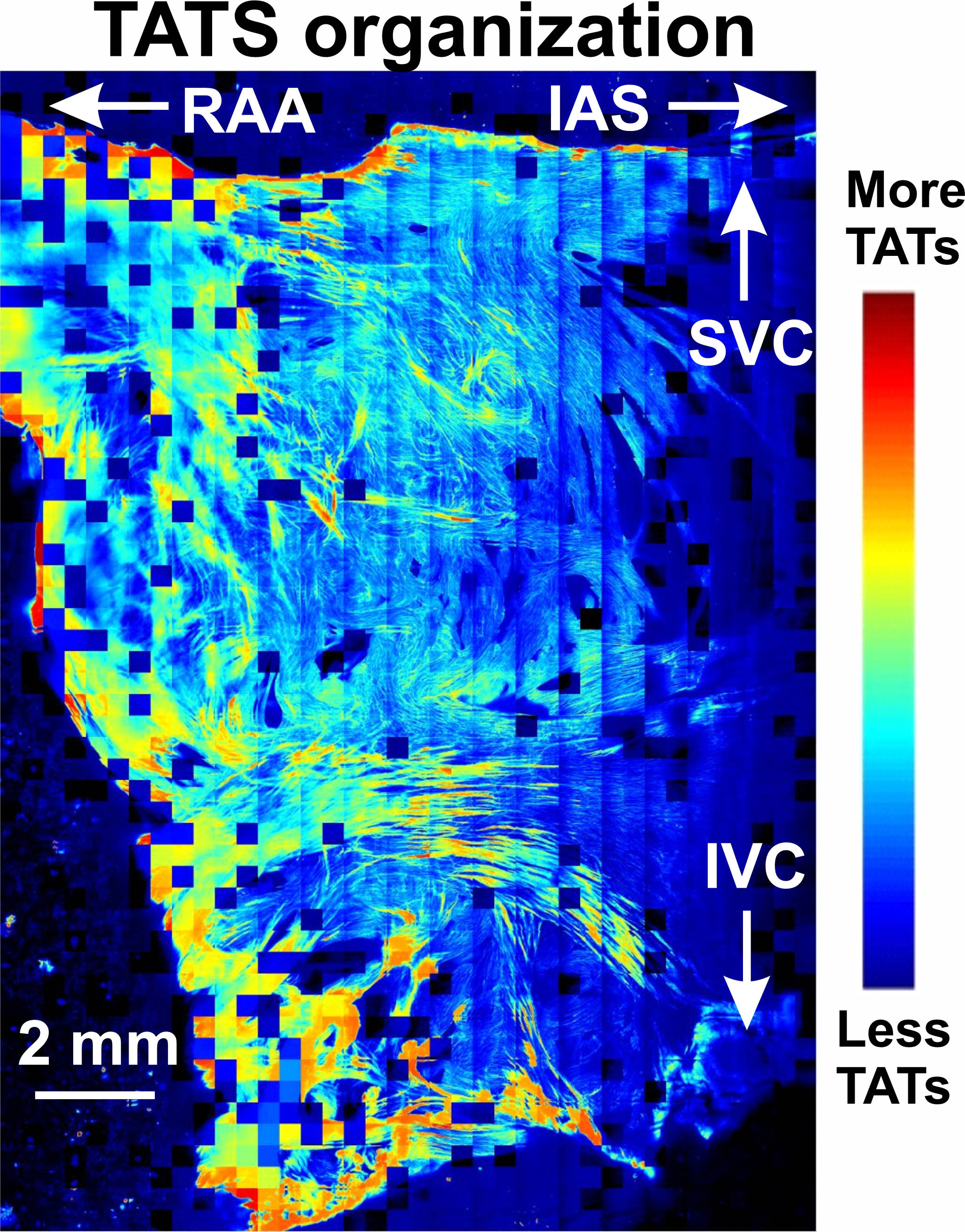
Then they compiled a color map of the entire right atrium, (Figure 3) finding that the right atrial appendage – the region furthest from the right ventricle, which has to do the most squeezing to move blood through the atrium into the ventricle – had the most T-tubules. The region closer to the right ventricle had fewer T-tubules. “That part is more like a breakwater, buffering the turbulence of the blood currents and helping the blood go into the ventricle,” said Dr. Lang.
He and his collaborators also conducted functional studies of the right atrium, showing that the electrical activity and calcium exchange levels varied dramatically between areas with few T-tubules and areas with many T-tubules. “These studies helped us connect specific cellular structures with their functions,” said Dr. Lang. “For the first time, we were able to see what the atrial cardiomyocytes look like in different places in the atrium.”
Dr. Lang also found that these differences in structure could be related to arrhythmias. For example, portions of the right atrium with fewer T-tubules appear to be more vulnerable to developing arrhythmias. That relative lack of T-tubules and consequent scarcity of calcium ion channels means that it’s harder for those cells to get rid of excess calcium, since they lack a robust network of pumps.
“There’s a lot of fantastic cardiovascular research going on, using traditional methods like studying different proteins and how they function in different diseases,” said Dr. Lang. “ My research incorporates my engineering background to develop platforms or methods that allow us to get information that we haven’t been able to get using traditional approaches.”
Identifying the Most Important Questions
Dr. Lang earned his bachelor’s and master’s degree in biomedical engineering from Zhejiang University in Hangzhou, China, then earned another master’s degree and doctoral degree in biomedical engineering at Washington University in St. Louis, Mo. He then completed a postdoctoral fellowship in pharmacology at UC Davis, working in the lab of Donald Bers, PhD, an expert in cardiac calcium signaling and cardiac electrophysiology. Dr. Lang then spent seven years as a research associate and scientist at the University of Wisconsin, Madison, before he was recruited to the UCSF faculty in 2022.
“I always believed that whatever I studied in biomedical engineering should eventual translate into clinical benefit for patients,” said Dr. Lang. “UCSF is a really great clinical and research institute, and I wanted to come to the frontlines of fighting different diseases. At UCSF, I get to work closely with the world’s best clinicians to figure out what are the most urgent questions to answer. That’s a really cool thing to do. Before, I was trying to choose between academia or industry, but I chose academia because I felt like you can touch the unknown. It’s not repetitive work that you do every day – you can push the edge of what’s known.”
“I am excited to have Dr. Lang join the Division,” said Jeffrey Olgin, MD, Ernest Gallo-Kanu Chatterjee Distinguished Professor in Clinical Cardiology and chief of the UCSF Division of Cardiology. “He is an outstanding researcher studying mechanisms of arrhythmias and how calcium dynamics affect arrhythmias. He has also been a leader in understanding how the sinus node regulates heart rate.”
At UCSF, Dr. Lang looks forward to building his lab and teaching trainees. “For me, teaching is a process of mutual growth,” he said. “When you teach, you learn things. It’s very inspiring when students ask questions and think in ways that are totally different from you. It’s always very exciting.”
He looks forward to continuing his investigations and starting new ones in partnership with other UCSF faculty. “It’s very joyful for me to develop my own research direction, and to combine my engineering background with biomedical questions,” said Dr. Lang. “I especially look forward to collaborating closely with clinicians who can help me smartly translate ideas into solid scientific questions.”
Outside of the lab, Dr. Lang enjoys hiking and spending time with his wife, Rong, an accountant, and their two young children.
- Elizabeth Chur